Lithium-sulfur batteries are batteries with lithium metal anodes and sulfur cathodes. They feature a high gravimetric energy density, although their volumetric energy density is limited due to the large amount of sulfur needed for the cathode. Lithium-sulfur has also been noted for potential cost reductions due to the abundance of sulfur, as well as enhanced safety due to the non-reactivity of sulfur. Lithium-sulfur is already seeing development across multiple continents and is expected to achieve mass production by 2033. IDTechEx predicts that by 2035, the lithium-sulfur market will exceed US$1.3 billion. For a year-by-year forecast broken down into application markets, see IDTechEx’s recent report: “Lithium Metal Batteries 2025-2035: Technology, Players and Forecasts“.
Degradation modes
Lithium-sulfur has seen development efforts in the past. However, the chemistry has been limited as a result of an intrinsic degradation method: polysulfide shuttle. Polysulfides of the form Li2Sx are produced in the cathode and shuttle into the electrolyte, effectively leaching active materials away. These polysulfides can also reach the anode and begin a cycle of their own redox reactions, which reduces the effective redox potential of the cell. Polysulfides can also form an insoluble layer of Li2S at the anode, preventing ion transport. The overall effect of polysulfide shuttle is to significantly reduce the coulombic efficiency of the cell, severely impacting battery lifetime.
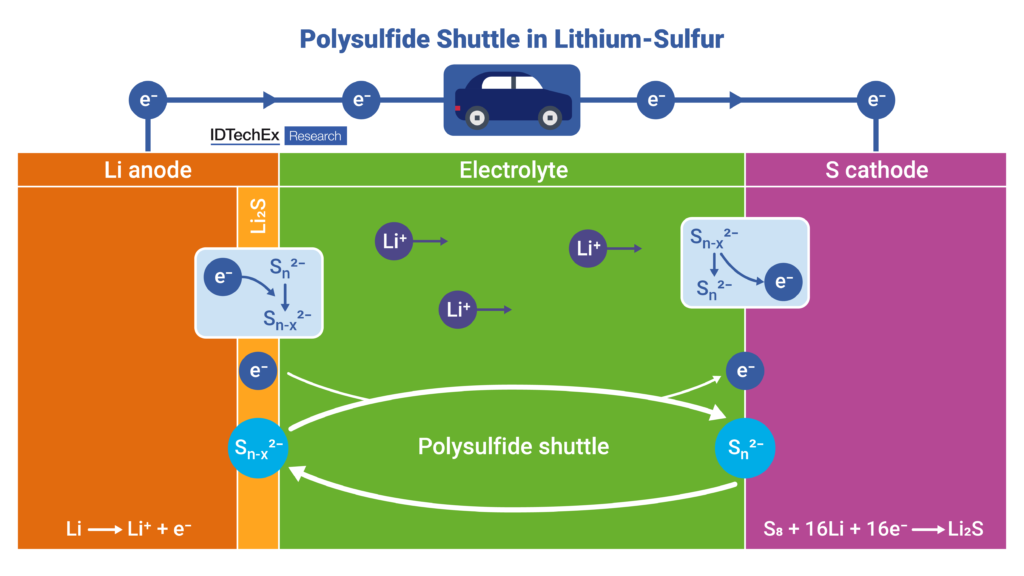
Lithium metal dendrite formation is also an issue, though it tends to be less significant than polysulfide shuttle. Lithium dendrites form at the anode and leach into the electrolyte, irreversibly reacting such that the active material of the cell is reduced. In addition, during charging and discharging, the sulfur cathode experiences significant swelling – as much as 80% during discharging. This places considerable pressure on the architecture of the cathode and potentially reduces the contact conductivity of the cell overall through the formation and nucleation of cracks.
Solutions to degradation
Polysulfide shuttle can be counteracted in several ways. The most obvious approach may be to use a solid electrolyte, as this prevents polysulfides from shuttling. However, this can lead to significantly reduced conductivity at the interface between electrolyte and cathode, as sulfur is already a poor conductor. Alternative liquid electrolytes are a more compelling option. Polysulfides are soluble in incumbent liquid electrolytes used in graphite-anode lithium-ion. However, there are other solutions in which polysulfides are not soluble, cyclic ethers, short-chain ethers and glycol ethers.
Alternatively, a separator layer/membrane may be used to prevent polysulfide shuttle. The chosen membrane must be selective, allowing lithium-ions to pass but not polysulfides. This could be achieved in one of three ways:
- Charge repulsion: utilizing the fact that polysulfides are negative and lithium ions are positive.
- Shielding: physically blocking ions from directly contacting active materials, preventing polysulfide leaching (e.g. using carbon nanotubes). As polysulfides are much larger than lithium ions, they can be selectively blocked.
- Adsorption: trapping polysulfides in the cathode using polysulfide-attracting materials. Micropores may be used for this method.
Cathode expansion can be solved using alternative cathode structures, e.g. expansion-tolerant lattices or stronger binders. Alternative materials may allow for the development of single-material structures without binders, which significantly enhances the rigidity of the current collector. An example is sulfurized polyacrylonitrile or SPAN.
Key takeaways
Lithium-sulfur has seen interest from major players, especially LG Chem, however they have since fallen quiet. Now, the industry is dominated by startups on the cusp of commercialization. Several examples are included below:
- Lyten started as a developer of 3D graphene materials but has since focused on the use of its materials for lithium-sulfur batteries. 3D graphene is used as a substrate for the sulfur cathode, which enables higher conductivity and reduces the effect of polysulfide shuttle. The company aims to commercialize for aviation and maritime applications by the end of 2025, and already has plans in the works for a gigafactory.
- theion is a German start-up developing sulfur cathodes formed from a pure sulfur wafer. The wafers are grown directly from molten sulfur. The technology remains in the development stage, however the company is exploiting ” battery testing AI platform to significantly reduce time-to-market.
- Li-S Energy is an Australian company working on lithium-sulfur. The company uses boron nitride nanotubes (BNNTs) to form its cathode structure. This allows for enhanced strength and conductivity. Li-S recently completed construction of a 2MWh pouch-cell production facility.
Lithium-sulfur’s higher specific energy but lower energy density make it particularly suitable for applications in aviation, defence and maritime, especially unmanned aerial vehicles (UAVs) or drones. However, the chemistry is expected to also see some deployment in electric vehicles, especially heavy-duty electric vehicles. IDTechEx predicts that by 2035, over 14 GWh will be produced globally. For an in-depth analysis of the market’s growth as well as player and technology assessment, see the recent report: “Lithium Metal Batteries 2025-2035: Technology, Players and Forecasts”.