Drones are becoming vital in modern industrial applications. Not only can they work in areas that humans would find difficult to access, but they do it at a significantly lower cost. For example, drones can inspect cables and pipelines over large distances that would typically require an expensive helicopter and crew. As technology progresses, these drones are filling new roles. Their low-cost, flexibility, and agility make them ideal for a wide range of applications, from parcel delivery to crop inspection and maintenance.
The versatility that makes drones so popular in so many settings is due to how they operate. Unlike large, military-style drones that use jet engines and propellers, most drones use rotors. These drones operate similarly to helicopters, creating lift through their rotors to produce a pressure difference (i.e., lower pressure above the blades and higher pressure below). Unlike helicopters, which have adjustable pitch, drone rotors are usually fixed in position. To remain stable, the rotor on one side of the drone usually turns in the same direction as its counterpart, which is diametrically opposite, and the other two rotors rotate in the opposing direction.
When it comes to their movement, drones use four primary controls: yaw, pitch, roll, and ascent/descent. Yaw rotates the drone clockwise or counterclockwise on its axis. Pitch tilts the drone forward or backward, causing it to move in those directions. Roll tilts the drone left or right. Lastly, ascent and descent control vertical movement, making the drone rise or fall. All these movements are achieved by adjusting the speed of the rotors.
For example, for a quadcopter to turn in a clockwise direction, the two rotors turning clockwise will speed up in comparison to the other two rotors. The larger the speed discrepancy, the faster the drone will rotate. These four basic movements can be combined to create highly agile drones. To dig deeper into how drones operate and the path to greater performance and efficiency, this blog explores how connectors impact drone design, including signal integrity, drone responsiveness, weight, and flight time.
Electric Motors
Each of the drone’s rotors is driven by its own electric motor. The most common type of electric motor chosen for this purpose is the brushless DC (BLDC) design because these motors provide very high torque for their weight and size. Additionally, their DC operation means they can operate directly from batteries, and their high efficiency means that those batteries last longer and encounter fewer thermal issues. Unlike competing motor designs, BLDC motors can function for a very long time at maximum torque, be precisely controlled, and operate over a wide range of speeds. Additionally, they are very robust and create less electrical noise than brushed motors.
The BLDC motor has a simple construction that only needs as little as three wires to operate. Like other types of motors, it has both a rotor and a stator. The rotor is a cylinder with permanent magnets embedded inside, while the stator has at least three poles constructed with electromagnets. With BLDC design, the motor turns due to the interaction of the magnetic fields from the rotor and stator. As the stator electromagnets are energized in sequence, the magnets on the rotor “follow” the rotating magnetic field. The stator can be constructed with more electromagnetic poles to create a higher torque motor but with lower speeds. To adjust the speed of the motor, the rotating field is quickened or slowed.
Controlling the Drone
The main parts of the circuit used to control the motor are the flight controller and electronic speed controller (Figure 1). The flight controller takes inputs from various sources and calculates the optimal speed for each rotor. These inputs can be internal, such as the sensors measuring the position of the rotors, or external, like the control signals from the operator. From this input, the flight controller outputs a signal dictating the speed of each rotor. The electronic speed controller (ESC) takes those control signals and delivers current from the battery to the electromagnets on the stator. The ESC can also include additional features, such as telemetry and active braking.
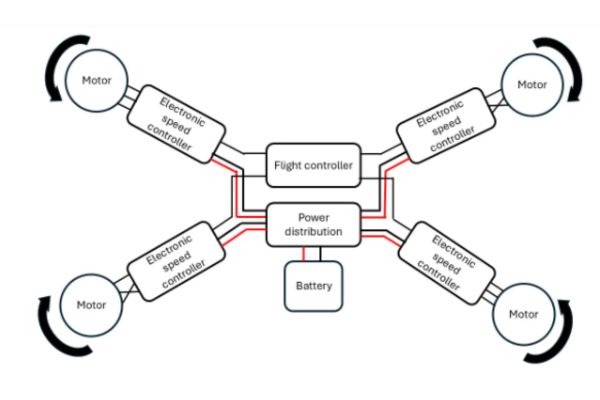
The conventional way for the flight controller to communicate with the electronic speed controller is by using pulse width modulation (PWM). In traditional PWM control, the duty cycle can vary between 100 percent on and 0 percent off with a set variety of steps between that can go as low as 1000μs. Today’s drones usually require a greater level of control than traditional PWM techniques can achieve, so faster communication protocols have been developed with less latency. For example, the OneShot ESC protocols offer faster switching times, with the quickest of these, OneShot42, having a pulse width time between 42μs and 48μs. Even this speed is insufficient for some applications, so the MultiShot ESC protocol was introduced, with an effective pulse width between 5μs and 25μs.
Traditionally, flight controllers use analog PWM signals to communicate with ESCs, but drone performance demands have increased and these signals have evolved. Hybrid protocols like OneShot and MultiShot reduce latency by refining PWM signals, but they still rely on analog transmission that falls victim to noise and signal degradation. The latest advancement is DShot, which delivers the control signals digitally. While DShot may not be as rapid as MultiShot, with a pulse rate of 6.7μs, it has better noise immunity and error checking, leading to greater overall accuracy.
Looking to the Future of Drone Design
As with almost all other electronic designs, customers are demanding smaller and more feature-packed drone designs that offer longer battery lives. With more drones in operation and the rise of semi- and fully autonomous models on the market, achieving more responsive control and lower latency operation will be fundamental to avoiding mid-air collisions. One of the key approaches to meeting these customer demands is through connectivity. In many designs, electromechanical components, such as connectors, can be the largest and heaviest devices in the design.
In most drone designs, the flight controller and the ESC are on different boards in order to fit into the required form factor, as well as to isolate the noise from fast current switching away from the delicate control circuitry. The ESC also needs to have a connection to each motor that can remain secure against vibration and other mechanical stresses. Connectors must accomplish this while delivering faster signals and higher currents reliably. These components also need to be as small and light as possible to create more compact designs and prolong battery life. Those demands will require connectors with traits such as those offered by the Positronic Dragonfly (DF), which can deliver both power and signals reliably in a small form factor (Figure 2).
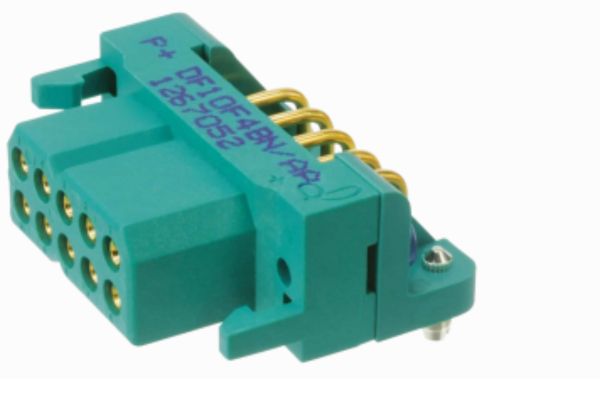
Connectors in the DF Series are built with high-reliability, high-retention contacts that have current handling capabilities of up to 20A per contact to provide the ideal solution for the demands of current and future drone designs.
Conclusion
In the evolution of drone technology, connectors play a pivotal role, ensuring reliable performance and enhanced efficiency. As drones become more integral to various industries, the demand for compact, lightweight, and high-performing connectors like the Positronic Dragonfly (DF) will continue to grow. These connectors not only support the intricate electrical needs of modern drones but also contribute to their overall agility and operational longevity. Embracing advanced connector solutions is essential for pushing the boundaries of drone capabilities and meeting the ever-increasing expectations of the market.
Author
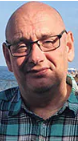
Since graduating with a BSc in Electronic Systems from the University of the West of Scotland in 1997, Alistair Winning has worked in electronics media across marketing, PR, and journalism roles. During that time, he worked as the editor of Electronics Engineering, Embedded Systems Europe, EENews Embedded, Technology First, Electronic Product Design and Test, and Panel Building and Systems Integration magazines. Currently, Allistair is the European Editor of Power Systems Design and a freelance writer, specializing in electronics and engineering.